AM Academy
AM Fundamentals Online Learning
Learn the most critical skills to navigate in the Additive Manufacturing market
AM Fundamentals
- Basic
- 49 minutes of on-demand videos
- 1-2 hours to complete
- 1 assessment
- Certificate upon successful completion
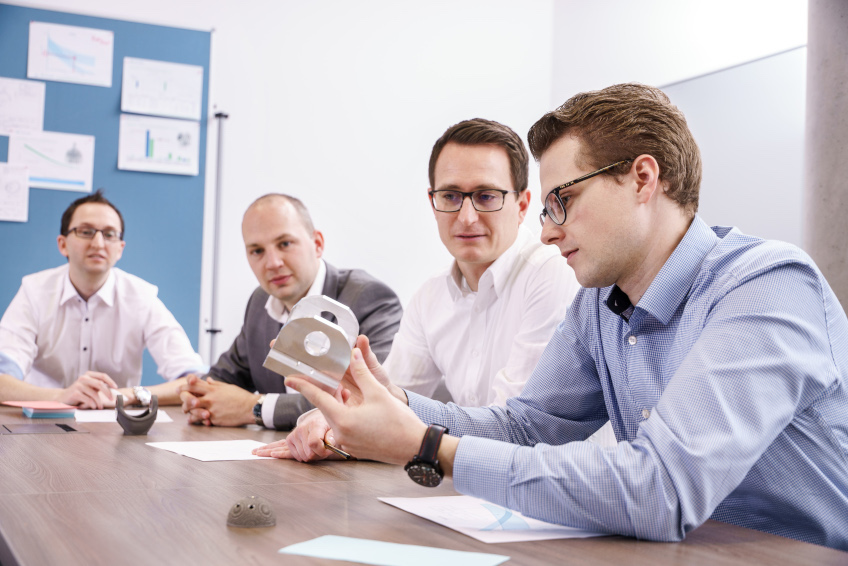